Empowering the grid: Virtual power plants
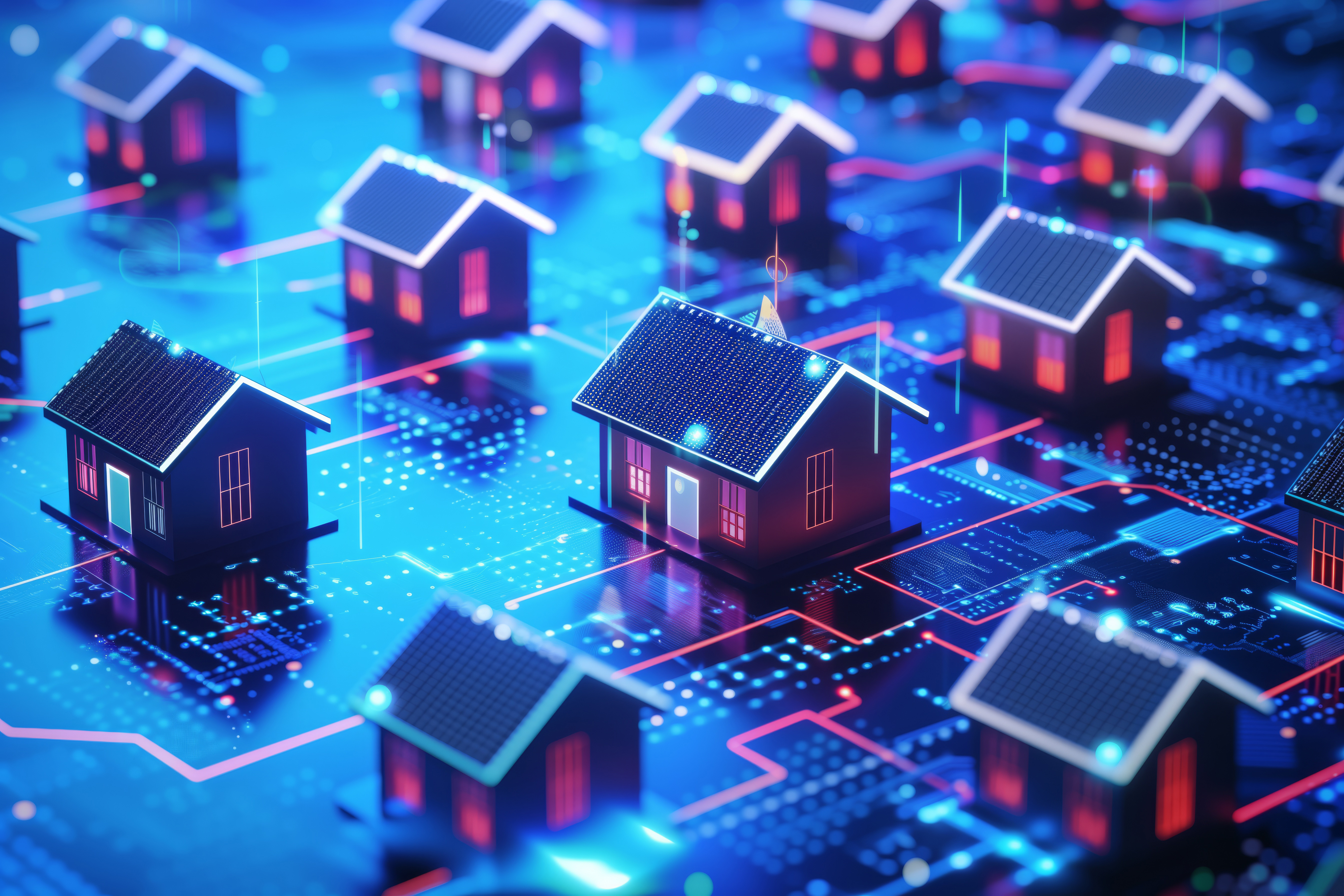
©Alina Zavhorodnii – stock.adobe.com
2024/09/25 – Virtual power plants are considered the key to aligning renewable energy with grid security. This is why forecasts predict an annual growth rate of about 38 percent over the next eight years. Virtual power plants leverage collective intelligence, combining decentralization with digitization, offering a modern and future-proof alternative to large centralized power plants, which are becoming less viable due to declining social acceptance.
A virtual power plant is a cloud-based network of decentralized units in the power grid, controlled by a central management system that enables communication between them. These units include power generators like biogas, wind, photovoltaic, and hydropower plants, along with energy storage systems, electricity consumers, and power-to-X plants, such as power-to-gas and power-to-heat. Power-to-X plants convert excess energy into gas or heat, which can be useful depending on demand. Even electric vehicles with bidirectional charging capabilities can be part of a virtual power plant. The main goal of a virtual power plant is to collectively market the electricity and flexibility that comes from aggregating these systems. Any unit that generates, stores, or consumes electricity can be part of a virtual power plant, provided it can communicate with other units.
At the heart of a virtual power plant is the central control system, which uses specialized algorithms to manage the elements as a single unit, responding to grid conditions and the requirements of transmission system operators. In combination with electricity trading, the virtual power plant can quickly and efficiently react to price signals in the market, adjusting its operations accordingly.
More efficiency through interaction and cooperation
Households or businesses often cite autonomy as their motivation for installing renewable power generation or energy storage systems. This mindset is understandable but doesn’t advance the energy transition. It’s comparable to early navigation systems: They could reliably direct you from point A to point B but lacked the ability to detect traffic issues and suggest alternative routes due to a lack of data exchange. It wasn’t until users became data generators, and central computers could aggregate this data in real-time, that navigation systems became capable of dynamically calculating the best routes. The willingness to interact and cooperate was essential to making complex systems like traffic more transparent, efficient, and beneficial for everyone. The same principle applies to the energy market.
This belief laid the foundation for the first ideas to connect individual systems into a virtual power plant. As early as the 1990s, initial theoretical concepts were developed to help tackle the challenges of the energy transition. However, limited computing and networking technology, as well as regulatory constraints, prevented widespread implementation.
It wasn’t until 2010 that virtual power plants could be realized on a larger scale. This was due to the availability of much more powerful computing, cloud, and AI technologies that enabled the creation of a robust, high-performance control system with real-time infrastructure. Additionally, in Germany, the federal government paved the way for virtual power plants by establishing the legal and economic framework for the energy transition through new electricity market designs and amendments to the Renewable Energy Act (EEG) in the wake of the nuclear phase-out. Similar developments were seen in other countries.
The virtual power plant operated by Norwegian state-owned company Statkraft was the first of its kind in Europe and remains the largest. In Germany, the company has already combined over 1,600 wind and solar plants with a total output of about 12,000 MW. In the United States, the Department of Energy estimates the current capacity of virtual power plants to be around 30 to 60 gigawatts. This represents about four to eight percent of the nation’s peak electricity demand.
Bright future prospects
With the elimination of technical and regulatory barriers, the rapid expansion of virtual power plants is within reach: The global market size is estimated at $1.86 billion in 2024 and is projected to grow to $23.98 billion by 2032, with an average annual growth rate of 37.70 percent. Europe dominated the market for virtual power plants in 2023, holding a share of 41.54 percent.
However, much of the projected growth is expected in other markets: China and the U.S. have committed to tripling their renewable energy capacities by 2030, aiming to replace fossil fuels in electricity generation and significantly reduce emissions from the energy sector. The increasing use of renewable energy will drive the need for virtual power plants to optimize the integration of various renewable sources like solar and wind into the grid, maximizing their efficiency and reliability. The U.S. Department of Energy aims to increase national capacity to between 80 and 160 GW by 2030, equivalent to 80 to 160 fossil fuel power plants that won’t need to be built.
Energy storage systems: The beating heart
Virtual power plants take on the role of a large power plant and can even match the installed capacity of nuclear plants. However, since renewable energy generation from sources like wind and solar is highly variable, energy storage systems play a crucial role. These systems allow surplus energy to be stored and released when needed, enhancing the flexibility of the entire plant. Especially during periods of low solar or wind production, storage is essential to maintain grid stability and the functionality of virtual power plants. After all, only as much electricity can be fed into the grid as is being consumed at any given time.
The importance of energy storage systems for virtual power plants is reflected in global investment decisions. In May 2019, the Asian Infrastructure Investment Bank (AIIB) granted $75 million for India’s Tata Cleantech Sustainable Infrastructure Project, with energy storage as one of the key investment areas. Similarly, in November 2019, the AIIB approved $100 million for the SUSI Asia Energy Transition Fund to provide equity financing for green energy solutions in Southeast Asia, including energy storage projects in North America and Australia. Increasing investments in the combination of renewable energy and storage are accelerating the growth of the virtual power plant market.
Incentives matter
For example, in 2023, Green Mountain Power, the largest utility in Vermont, offered customers the option to lease or buy a large battery at a discounted price. There was also support of up to $10,500 if customers agreed to share the stored energy with the utility. According to the Vermont Public Utility Commission, this also ensures backup power during outages.
The right mix
Alongside storage systems, a mix of energy generation sources in a virtual power plant is crucial for balancing the energy supply. Therefore, the portfolio of a virtual power plant includes not only variable sources like wind and solar but also controllable ones such as biogas and hydropower (run-of-river and pumped storage plants), as well as flexible electricity consumers and power-to-X systems. All these units can buffer fluctuations in energy supply caused by low wind or cloud cover, both positively and negatively.
Electric vehicles with bidirectional charging capabilities could also play an important role in the future. The theoretical storage capacity of one million connected, unused electric vehicles at night is about 50 gigawatts—roughly equivalent to the energy lost through Germany's phase-out of coal and nuclear power by 2038.
More flexibility in the electricity market
The strength of virtual power plants lies in their flexibility. They can dynamically adjust their output to market conditions, allowing them to respond to fluctuations in electricity prices. This makes them superior to traditional large power plants, which are often slower to react to changes. For instance, a virtual power plant can quickly reduce the output of biogas or hydropower plants when there is a surplus of wind energy, and conversely increase production during energy shortages. The commands to increase or reduce output are transmitted by the control system via IT interfaces (API) or remote-control units installed at the plants.
Flexibility also has an economic dimension: The price of electricity changes up to a hundred times a day in the intraday market at the power exchange, and double- or triple-digit price spreads per megawatt-hour are common. With their aggregated output, virtual power plants can follow price trends at the power exchange, offering their electricity particularly efficiently on the market. In contrast, large power plants, designed for consistent output, quickly hit their technical limits. For example, the response speed of a lignite power plant is akin to a large container ship with considerable "braking distance," before it can react to increased wind generation by throttling its turbines. The absurd consequence is that, without virtual power plants, climate-neutral wind farms must be temporarily disconnected from the grid to prevent overloads. In 2023, this "redispatch" effort alone cost Germany €4.2 billion—a cost borne by consumers.
Interfaces and network connectivity
The transmission of control commands and data within a virtual power plant takes place between the highly secure, redundant control system and the facilities via specially secured data connections. These connections use public communication infrastructure but are shielded in terms of their protocols from other data traffic.
The control system of a virtual power plant continuously communicates with individual units via secure data connections. This bidirectional communication enables not only the transmission of control commands but also the collection of real-time data for monitoring production and consumption. These data are crucial for forecasting and optimizing deployment planning and power trading. Much of this evaluation is automated, with the virtual power plant’s software architecture handling key tasks in initiating and executing trades at the power exchange.
Another possibility: Digital twins of virtual power plants could be created, allowing electricity grids to be simulated in real time and in great detail when needed. This could enable consumers to know at a click whether a grid connection is possible at their location. Using historical and current measurement data from local grids, weather data, and socioeconomic analyses, it would also be possible to estimate where further grid expansion would be efficient—and when and where fluctuations in the grid might be expected.
Balancing power from virtual power plants
A significant advantage of virtual power plants lies in their provision of balancing power, which is used to compensate for short-term fluctuations in the power grid and stabilize grid frequency. Balancing power is categorized as positive—when energy is fed into the grid to cover shortages—and negative—when energy production is reduced to handle a surplus. To offer balancing power, a power generation unit must have at least one megawatt of capacity (previously five megawatts). Smaller plants can only meet this threshold by pooling together in a virtual power plant: The aggregated power plant cluster can thus handle larger balancing power requests from transmission system operators, and the revenues from balancing power sales are shared. Generation units like biogas plants and hydropower stations, as well as large energy storage systems, can respond flexibly. They can not only throttle or halt production during electricity oversupply (negative balancing power) but also feed additional electricity into the grid during shortages (positive balancing power).
Statkraft became the first company in Germany to participate in the balancing power market with a wind farm pool in early 2016.
Electricity consumers in virtual power plants
Even electricity consumers can offer negative balancing power: For instance, an industrial facility connected to a virtual power plant can receive a command from the control center to ramp up production and draw excess electricity from the grid. Electricity can be consumed precisely when it is abundant and cheap on the market. A production facility that shifts its largest electricity consumption to these low-cost periods could save up to a third of its electricity costs.
Once again, this is impossible without digitization and data exchange. For companies with their own EV fleets and PV systems to flexibly switch between their roles as consumers or electricity providers, both the identities of their PV systems or vehicles and their respective consumption and generation data must be digitally captured and linked. Otherwise, it will be unclear which device is feeding how much green electricity into the grid. Currently, there are no digital registers that contain this information.
Integration into households
While industrial consumers are already benefiting from virtual power plants, household integration is still dependent on the rollout of smart meters. In Scandinavian countries, intelligent electricity meters are already present in virtually all homes. In many other European countries like Spain or Italy, the adoption rate is well above 80 percent.
In Germany, however, less than one percent of households are equipped with smart meters. Many homes still use rotary meters from the 1920s. Only once these are replaced—expected to happen by 2032—will it be possible to control household appliances such as washing machines, water heaters, or dishwashers cost-effectively, aligning electricity consumption more closely with market conditions. Likewise, PV systems, balcony power plants, or electric cars at home charging stations can only become part of a virtual power plant with a smart meter.
The benefits for households can be substantial: For example, the central algorithm might decide it’s cheaper to charge an electric car to only 80 percent, using the remaining 20 percent as flexibility to stabilize the grid—and in return, consumers could enjoy lower electricity rates. By the end of 2025, consumers are expected to benefit from dynamic electricity tariffs that enable such models.
Virtual power plants digitize the energy sector
Virtual power plants represent the digital transformation of the energy sector. Like other societal systems, electricity supply is undergoing a structural shift: Virtual power plants symbolize the transition to decentralized, networked, and flexible energy supply—moving away from large fossil or nuclear power plants. Through their hybrid approach, which integrates various energy sources, storage systems, and generation technologies with data-driven control, they are well-prepared for the future demands of electricity markets. Similar to hotel platforms that own no hotels, or car-sharing platforms with no fleet of their own, virtual power plants contribute to the democratization of energy supply. At a time when both transportation and digital infrastructure are becoming increasingly electrified, virtual power plants offer a sustainable and efficient solution for the future of energy.