Energy storage for the energy transition – but which technology?
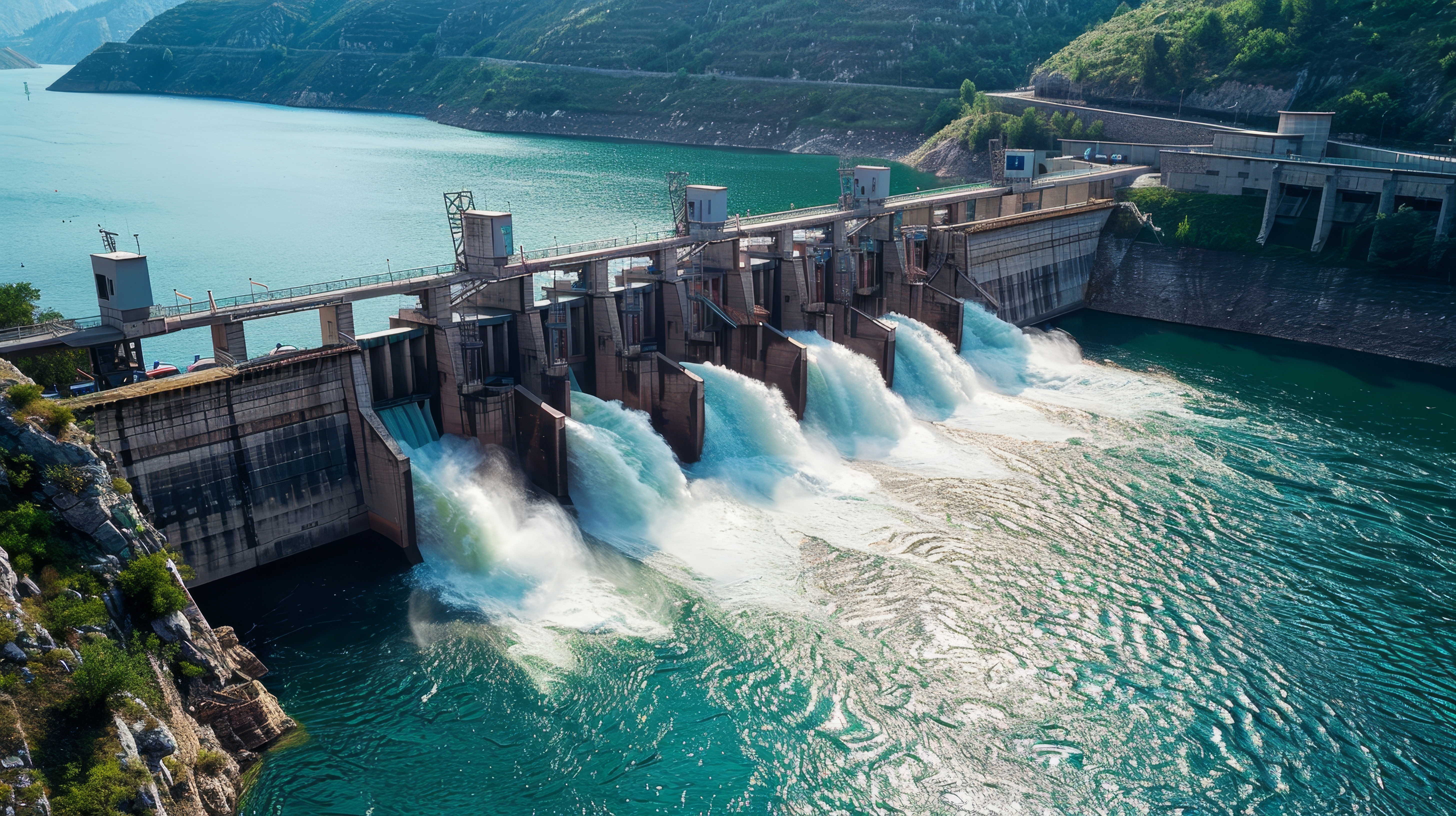
(©songwut – stock.adobe.com)
2024/06/27 – It is now widely accepted that storage technologies are indispensable for the expansion of renewable energies. In numbers: Fraunhofer ISE quantifies the potential of stationary battery storage in Germany alone for the year 2040 at about 140 gigawatt-hours – this corresponds to the capacity of all energy storage systems sold worldwide in 2022.
But which storage technology is most helpful in achieving the expansion goals of renewable energies? The range of existing storage technologies is at least as broad as the methods of electricity generation. We have listed and compared the most important technologies:
In principle, there are five different storage systems:
- Mechanical energy storage
- Chemical energy storage
- Electrochemical energy storage
- Electrical energy storage
- Thermal energy storage
Mechanical Energy Storage:
Mechanical energy storage includes pumped storage, compressed air storage, and flywheel storage.
Pumped Storage Power Plants: Pumped storage plants are the most widespread form of energy storage (worldwide share about 96%). They have been in use since the 1920s and utilize excess electrical energy to pump water from a lower reservoir to a higher one. When electricity is needed, the stored water is released back into the lower reservoir, driving turbines connected to generators. These convert the kinetic energy of the water back into electrical energy. Pumped storage is very useful for load management in power grids due to its high capacity and efficiency and its ability to quickly provide large amounts of power. The efficiency of a pumped storage power plant is about 70%. In Germany, around 7 GW of electrical capacity from pumped storage power plants is installed. The world's largest power plant of this type is located in Fengning, China, with a capacity of 36 GW.
Although pumped storage power plants are currently the most widely used energy storage technology, from an ecological point of view, their construction involves a massive and irreversible intervention in the landscape – and is only possible with appropriate topographical conditions such as height differences between 70 and 600 meters. The greater the height difference, the more efficient the plant.
Compressed Air Energy Storage (CAES): Compressed air energy storage systems store energy by compressing air in underground containers or natural formations such as salt caverns using excess electricity. When electricity is needed, the compressed air is released and passed through a turbine that drives a generator. Compressed air storage can be implemented on a large scale and offers a cost-effective means of long-term energy storage. Some modern CAES systems utilize heat generated during the compression process to further increase efficiency.
A key feature of a compressed air storage power plant – like other peak-load and storage power plants – is that it can be started very quickly. For example, in Huntorf near Elsfleth, Lower Saxony, 50% of the output is available within three minutes, and 100% after about ten minutes. The efficiency is about 65-70%.
Flywheel Storage: Flywheel storage systems store energy in the form of rotation. A flywheel is set in motion with excess electricity and kept at high speed in a nearly frictionless vacuum environment using magnetic bearings. When electricity is needed, the rotational energy is used to generate electricity through an electric generator. Flywheel systems can respond very quickly, making them particularly suitable for applications requiring very rapid energy delivery – such as stabilizing grid quality or frequency regulation. The efficiency of flywheel storage is relatively high at 70%, but idle losses of about 20% per hour must be considered.
Beacon Power operates a flywheel storage power plant in Stephentown, near New York, with 200 flywheels, collectively providing a capacity of 5 MWh and a power of 20 MW. The units operate at up to 15,000 revolutions per minute. The rotors of the flywheels are made of wound CFK fibers cast with resin. The storage park serves for frequency regulation, and the service is sold to the New York power grid.
Chemical Energy Storage:
Chemical energy storage includes Power-to-Gas, Power-to-Liquid, and Power-to-Chemicals systems.
Power-to-Gas (PtG): Power-to-Gas refers to converting excess electricity into hydrogen or methane, which can be used as gaseous fuels. The process typically starts with water electrolysis, where hydrogen (H₂) and oxygen (O) are split from water using electricity. The hydrogen can either be stored or further used. In an additional step, the generated hydrogen can be converted to methane (CH₄) by combining it with carbon dioxide (CO₂). This synthetic methane can be fed into the existing natural gas network or used in other sectors such as mobility or industry.
A significant advantage of Power-to-Gas storage is that energy can be stored long-term and almost loss-free. However, the efficiency is relatively low at 30-50%.
Power-to-Liquid (PtL): Power-to-Liquid focuses on producing liquid fuels such as diesel, kerosene, or methanol using excess electrical energy. The basic process is similar to Power-to-Gas, where hydrogen is first produced by electrolysis. In the Fischer-Tropsch synthesis or methanol synthesis, the generated hydrogen is combined with CO₂ and converted into liquid fuels through chemical processes. These fuels can be used as sustainable alternatives to fossil fuels and help reduce CO₂ emissions in sectors like aviation and shipping.
Power-to-Chemicals (PtC): Power-to-Chemicals refers to converting electrical energy into valuable chemicals or raw materials. This includes a wide range of products depending on the processes and target chemicals used. For example, hydrogen produced by electrolysis can be used to manufacture ammonia, which plays a key role in fertilizer production. Other chemicals that can be produced through electro-synthesis include alcohols, acids, and plastic precursors.
Electrochemical Energy Storage:
Electrochemical energy storage includes conventional battery storage as well as redox or hybrid flow batteries.
Conventional Battery Storage: Conventional batteries, such as lithium-ion batteries, are used in many electronic devices, electric vehicles, and as stationary energy storage. These batteries store energy through electrochemical reactions between two electrodes – an anode and a cathode – separated by an electrolyte. During charging, the anode absorbs electrons while ions migrate from the cathode to the anode through the electrolyte. During discharge, the anode releases electrons that flow through an external circuit to the cathode, while the ions return to the cathode. This electron movement generates an electric current that can be used.
Conventional battery storage has a relatively high efficiency of 65-80%. They can also store electrical energy for a long time and release it relatively quickly.
Redox Flow Batteries: Redox flow batteries store energy in chemical compounds. Stored in solutions, these compounds circulate through cells where the electrodes are separated by a membrane. In redox flow batteries, oxidation and reduction reactions (redox reactions) occur in separate containers. These containers are connected to electrodes separated by an ion-permeable membrane. Electrolyte solutions are pumped through the cell from their respective containers. During charging, the dissolved active species are reduced and oxidized; during discharge, the process is reversed, generating electricity. Redox flow batteries are currently used, among other things, as a backup source for mobile phone base stations or buffer batteries for wind turbines.
Hybrid Flow Batteries: Hybrid flow batteries combine elements of conventional solid-state batteries with those of redox flow batteries. In these batteries, one of the electrodes is solid, while the other electrode uses a dissolved active species in a liquid. The solid electrode can be a typical battery electrode (like in lithium-ion batteries), and the liquid electrode functions similarly to a redox flow battery. This configuration allows the advantages of both technologies to be utilized, such as the higher energy density of solid-state batteries and the greater scalability and easier maintenance of flow batteries.
Electrical Energy Storage:
Electrical energy storage includes superconducting magnetic storage and supercapacitors.
Superconducting Magnetic Energy Storage (SMES): Superconducting magnetic energy storage systems store energy in the form of a magnetic field generated by a current flowing through a superconducting wire. Superconductors are materials that can conduct electric current without resistance below a specific critical temperature. When energy is needed, the current flow from the coil is released. The current can then be used to supply electrical loads. The fast response time of SMES systems makes them ideal for applications that require rapid energy release, such as stabilizing power grids during sudden load changes. However, commercial applications are not yet known.
Supercapacitors: Supercapacitors have very high capacitance values and can store and release energy very quickly. They use electrodes typically made of highly porous carbon-based materials and a liquid electrolyte. The high porosity of the electrodes increases the effective surface area and allows for a greater accumulation of charge. Unlike batteries, which rely on chemical reactions, supercapacitors store electrical charge physically on the electrode material's surface. When a voltage is applied, ions from the electrolyte migrate to the oppositely charged electrodes, leading to a very rapid charge. During discharge, the ions return to the electrolyte, releasing the stored electrical energy almost instantaneously. This makes supercapacitors particularly useful for applications requiring short-term high power, such as vehicle acceleration or peak shaving in power grids.
The efficiency of supercapacitors is between 85 and 98%. However, the self-discharge rate of 14% per month speaks against long-term energy storage. Supercapacitors are already used in smartphones and laptops to support lithium-ion batteries when power demand needs to be met very quickly. However, their significantly lower energy density currently argues against commercialization for other applications. This means they store less energy per unit volume or weight. For this reason, they are currently not suitable as the sole energy storage for applications requiring a high amount of energy in a small space, such as in mobile phones or electric cars.
Thermal Energy Storage:
Thermal energy storage includes sensible heat storage, latent heat storage, and thermochemical storage. They are primarily used in the building sector for heating but can also be used for electricity storage and production.
Sensible Heat Storage: Sensible heat storage uses the ability of materials to store heat by changing their temperature. The stored thermal energy is directly proportional to the temperature change of the storage medium. Typical materials for sensible heat storage are water, sand, or rock. When excess thermal energy is available, it is used to heat the storage medium. The stored energy is later released by cooling the medium, with the released heat used for heating or electricity generation.
Popular applications for sensible heat storage are hot water tanks for solar thermal systems as buffers for building heating.
Latent Heat Storage: Latent heat storage utilizes the energy absorbed or released during the phase change of a material (e.g., from solid to liquid). This energy is referred to as latent heat. Phase change materials (PCMs) such as paraffins, salt hydrates, or metal alloys are common storage media. When heat is supplied, the PCM melts, absorbing large amounts of energy. This energy remains stored until the material solidifies again, releasing the stored heat. This process allows for a very high energy density compared to sensible storage and a nearly constant temperature during the phase change.
A widespread and particularly appreciated application in the cold season: reusable hand warmers in pocket format use latent storage. A new application area is the use in electric vehicles. Here, the necessary heating power for the interior can be provided by the thermal storage instead of the traction battery, which can improve the range of electric vehicles in winter.
Thermochemical Storage: Thermochemical storage uses chemical reactions to store and release heat. This technology offers the potential for almost loss-free long-term heat storage. During storage, heat is used to trigger a reversible chemical reaction that produces reaction products that store energy in chemical form. To release the stored energy, the reverse reaction is triggered, converting the stored chemical energy back into heat. The main advantage of thermochemical storage lies in its ability to store heat over long periods without significant energy losses and provide it in a usable form when needed.
Conclusion:
The use of different storage technologies depends on various application fields in conjunction with the technological properties required. Technological properties such as storage duration (short-term or long-term storage), power (in MW), and capacity (in MWh) determine whether a particular technology is suitable for different applications. Studies examine the application fields at different levels: storage application fields include self-consumption optimization, frequency regulation, and peak shaving. Application fields can also refer to different energy sectors. The World Energy Council associates the characteristics of different battery technologies with various application cases. A schematic overview that relates storage size to charging and discharging times shows the energy storage technologies and potential business models suitable for them:
The result: Lithium-ion battery storage is currently suitable for almost all applications due to its high maturity, long lifespan, scalability, and fast charging and discharging capabilities, and is generally superior to other storage technologies. They are already widespread today and, due to the dynamic development of electromobility, are increasingly being used for grid stabilization.